Natural disasters such as, the July 2010 floods in Pakistan,1 and during late December 2010 and early January 2011 in Queensland,2 prove how easy it is for unpredictable weather patterns that may be associated with climate change to raze buildings and devastate communities. Perversely, architecture contributes significantly to the problem, being responsible for 40 per cent of the urban carbon footprint,3 where the burning of fossil fuels for the domestic technologies housed in buildings has occurred on an industrial scale and has produced a toxic relationship between the production of architecture and its impact on an environment.4
When communities devastated by natural disasters are rebuilt, the basic approaches used to reconstruct them are essentially the same as those used to create these vulnerable buildings in the first place. The current emphasis on remedial interventions is to build physical barriers that intervene between architecture and the elements; in other words, to isolate human habitation from nature. The effectiveness of these barricades depends on our ability to future scope changes in the environment. For example, when the Thames Barrier was designed in the 1970s, global average sea levels were rising at about 1.8 millimetres a year and global warming was not seen as a threat. In the past fifteen years the rate has nearly doubled to about 3.1mm a year, and many scientists expect it to accelerate still further, which will require the barrier to be redesigned and rebuilt.5 In the coming decades there will be further unprecedented variations in weather patterns associated with global warming, and by 2050 more than two-thirds of us will be living in urban environments, which will amplify the impact of climate change on urban communities.
While prophylactic construction measures could mitigate the impact of environmental disasters (such as providing communities with floating homes in flood ravaged districts, or relocating houses from the surface to subterranean dwellings in hurricane prone areas) it is also essential to generate innovative construction methods that produce buildings which can respond in real time to more subtle environmental changes so that buildings are constantly adapted to their surroundings. The prospect of a built environment and construction industry that is sensitive and connected to its environment, rather than separate from it, offers new approaches to sustainable development.6 Sustainable architecture could therefore be regarded as building interventions that are integrated into the biosphere where they engage in evolvable cycles of material exchange with their surroundings. Under these conditions, the construction of architecture has the potential to make a positive impact on the urban environment through the tuning of interfaces coated with materials that respond to chemical fluctuation in their surroundings. Since the built environment constitutes two per cent of the Earth’s surface,7 the linking of architecture with natural systems presents a significant potential and scale of operation that may assist in combating climate change. Indeed, Stephen Chu has already observed the potential for making a difference to global warming in his recommendations to paint all the reflective surfaces of buildings white, to prevent passive heating through solar radiation.8 If this passive change alone radiates enough heat to cause a 44 billion tonne reduction in greenhouse gas emissions (a calculation made in collaboration with Art Rosenfeld from the Californian Energy Commission, using data based on the reflective surfaces available on the ten largest cities9), then it could be expected that active surfaces would make an even greater contribution to combating climate change.
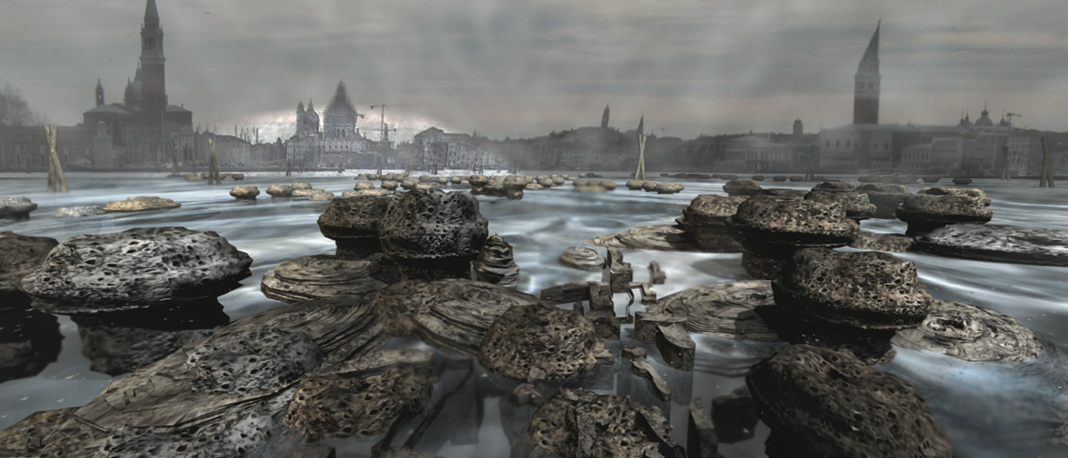
‘Venice Reclaimed’ Progressive accretion of an artificial limestone reef underneath the city of Venice through the collective action of programmable protocells spreads the point load of the city which currently rests on wood piles over a broader base and attenuates sinking. Drawing by Christian Kerrigan
Visionary architect Neil Spiller condemns modern building processes as ‘stupid’ as they are based on Victorian ideologies that employ inert and unresponsive building materials that render them belligerent to the continually changing urban environment.10 They are unable to respond to the smallest changes in their surroundings, let alone possess any capacity to respond meaningfully to more dramatic changes. In a constantly challenging and changing environment, responsive building materials are needed – materials which have flexible and robust properties that are contingent on their exact circumstances. The material of choice is biology, which has often been used as fabric for architectural practice, as in the case of the living bridges of Cherrapungi, which are created from the trained roots of trees that stretch to more than 30 metres, can support the weight of 50 people, and take 15 years to grow. In an industrial age, ‘natural’ biology has too many limitations to use in construction. To create more life-like buildings, by looking for the ‘drivers’ of biology architects have sought materials that retain the uniquely adaptable characteristics of biological systems. They have sought materials that fall between traditional durable materials and living systems.
While R. Buckminster Fuller supposed that these drivers were mathematical and sought solutions using digital computation to explore generative forces, Antonio Gaudi explored the physical and chemical imperatives of materials through his unique architectural style. To conduct his experiments, Gaudi created a set of unique and individually crafted elemental forms by suspending clay in hanging cloths during the construction of La Sagrada Familia. He let the physical forces of gravity shape the material. These material, self-organising imperatives were then used to generate his unique style of ‘organic’ design. Unlike most architecture, which normally follows a top-down blueprint, Gaudi controversially assembled the architectural components using a bottom-up approach. Rather than impose his own personal inclinations, Gaudi allowed the rules of gravity to generate the cathedral’s design, so creating his hallmark aesthetic style.
In a more contemporary setting, artist Roger Hiorns has also employed a ‘bottom up’ approach to architectural construction, using the intrinsic growth imperative of copper sulphate crystals. Using reinforced steel, Hiorns sealed off a derelict building and filled the interior with a boiling supersaturated solution of salt. He then left the construction site to self-organise, and drained the building after two weeks. The final violet-blue crystal studded appearance of Hiorns’ unique architectural interior is not generated by the instructions specified by a blueprint. Yet it offers a repeatable method of construction for the production of an architectural interior. Strikingly, large crystals appear on the surfaces of glass and enamel in the derelict building. The crystals are chemically responding to the smooth surfaces of these materials which facilitate crystal growth, yet poetically suggest that the agents are able to identify man-made objects in the landscape.
My research explores the material applications of a new group of technologies with life-like qualities called Living Technology.11 These technologies, which may be thought of as ‘Living materials’, exhibit some of the properties of living matter and may provide a new generation of smart materials that exist somewhere between ‘natural’ biology and traditional, inert building materials. Living materials therefore possess the environmental responsiveness and contingency of biological systems without actually being ‘alive’. They offer architects a potential interface through which they can respond to new challenges. Living materials do not rely on metaphor, analogy, human interface or mimicry for interpretation. Instead they work directly with the living processes, such as movement, sensitivity, self-organisation and interaction between populations of embodied, complex agents.12
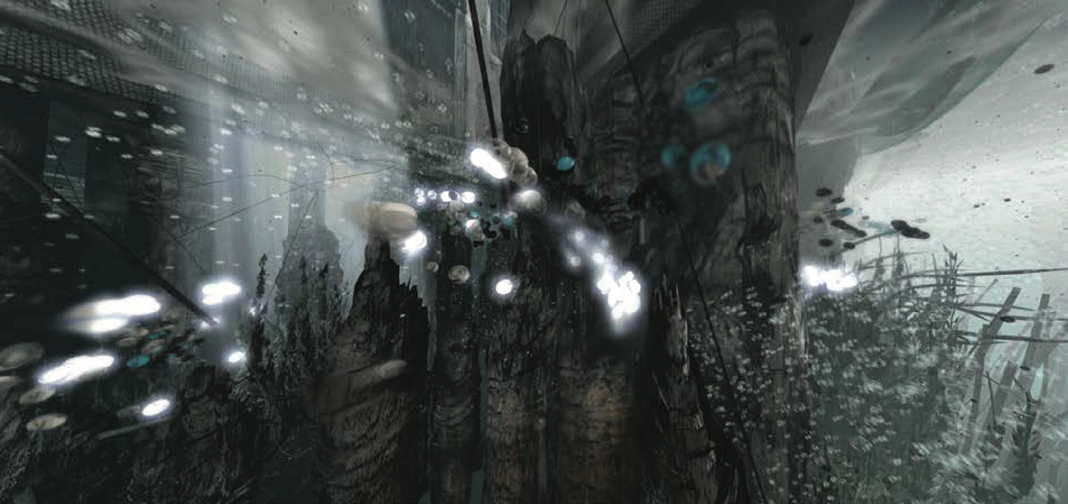
‘Protocell Generated Artificial Limestone Reef’. Protocells are programmed to move away from the light filled canals and towards the darkened foundations of the city, which rest on woodpiles. Here the protocells produce a limestone-like shell, which accretes with time and eventually produce an artificial reef underneath the foundations of the city, which spreads its point load attenuating the sinking into the soft delta soils on which Venice was founded. Drawing by Christian Kerrigan
Living materials offer new opportunities in sustainable development, since they are in constant conversation with the natural world through sets of chemical reactions called metabolism. A metabolism is the chemical conversion of one group of substances into another, either through the production or the absorption of energy, which enables Living materials to make the most of their local resources in an adaptable and sustainable way, in a similar manner to biological systems. Since Living materials are a technology, they can be designed to perform a specific function, or range of functions, and may be thought of as part of the spectrum of the new science of synthetic biology, which broadly engages with the ‘rational engineering’ of living systems. Typically, this is carried out via a top-down approach, where biological cell systems are hacked into in order to change their function or make them perform more efficiently in some way. This usually involves genetic modification of organisms, is expensive, difficult to do and heavily regulated.
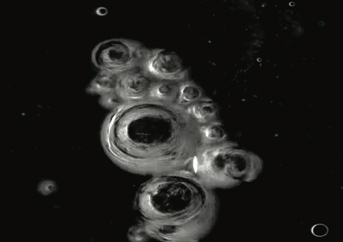
‘Protocell Roses’ Protocells are dynamic systems which exhibit emergent life-like qualities resulting from the chemical activity at an oil/water interface. These droplets are able to distribute materials over space and time producing complex microstructures that sometimes resemble biological structures. Photograph Rachel Armstrong
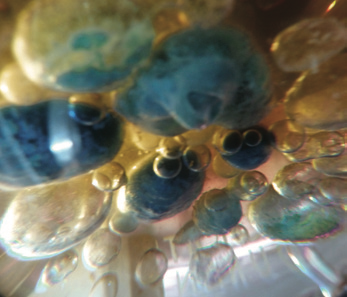
‘Protocell organs’ These protocells were engineered for Hylozoic Ground, a living installation by architect Philip Beesley for the Venice Architecture Biennale 2010. They functioned as chemical organs that responded to carbon dioxide produced by gallery visitors and caused the protocell metabolisms to create crystalline precipitates, which indicated the presence of this waste respiratory gas in the environment. Photograph Rachel Armstrong
Top-down modification is not exclusive to genetic engineering, but can also involve other forms of cell modification, such as the magnetic manipulation of the cytoplasm of green algae Bryopsis plumosa. This seaweed has a powerful ability to regenerate following mechanical destruction, and in the repair stage is able to take up foreign particles into its cell body. Exploiting its extraordinary ability for self-repair, this green algae can be grown into a specific shape by adding magnetic particles to the culture medium and allowing the cell body to regenerate under the influence of magnets. The outcome is that the regenerating plant takes on the shape of the magnetic field in which the plant reconstitutes itself. A speculative application of this extreme form of morphological design could be used to naturally protect the volume of sand on popular beaches. Bryopsis could be grown into the shape of groynes, using hydroelectrically powered magnets to shape the development of the algal bloom which is greatest in the summer when erosion is undesirable, and is absent in the winter when it is desirable for sand to be deposited on to the shore, creating a seasonally active architecture to protect valuable coastlines.
A bottom-up approach to synthetic biology is also possible from the most basic elements that constitute living systems or artificial biologies.13 An example is a dynamic oil in water droplet system, or protocell. These programmable agents act as slow release containers that are able to distribute materials in time and space. Their local responses are triggered by local environmental conditions. Since bottom-up synthetic biologies, or artificial biologies, are not technically ‘alive’ (as they do not use DNA/ RNA), they constitute a new group of materials that may be explored in the context of a built environment being able to act simultaneously as environmental monitors and effectors. For example, protocells can produce insoluble crystals of ‘carbonate’ from dissolved carbon dioxide that produces a shell-like structure as a result of the chemical programming inside the protocell species when it comes in contact with the carbon dioxide in the water. A variety of different salts can be used to create these carbonate shells, and can be chemically programmed by selecting the environmental conditions or the metabolism of the agent to produce crystals of different colours and textures for synthetic ‘carbonate shells’.
The ability to program observable results within a protocell system enables environmental processes that are otherwise unseen to be viewed, without having to employ advanced imaging techniques. These artificial biologies then can act as an early warning system for environmental change. However, artificial biology can perform parallel processing and it is possible to select metabolisms that both act as monitors of change and can simultaneously make a remedial intervention. For example, if a calcium salt is used to program the behaviour of protocells, then when the presence of carbon dioxide is detected and visualised it is used to produce artificially generated, crystal thick shells that are made from calcium carbonate. Calcium carbonate is a limestone-like substance which can be employed strategically to serve as a building material. Limestone has been used as a building material since historic times and is created by the accretion of tiny shells of fossilised marine life.
Using the protocell system, it is possible to think of how the accretion process that has constituted the production of limestone could be artificially extended so that this natural building material continues to grow, self-repair, and even respond to changes in the environment in real time. To do this, the movement of the protocell can be directed by chemical gradients or light sensitivity. The rational engineering of this simple system in this context offers a technological interface that is embedded in the natural environment where it possesses a meaningful role relevant to humans, and even to wildlife.
While conventional building projects are constructed in anticipation of future needs, Living materials provide real time remediation through the interface of responsive materials, such as those that are being engineered with the protocell system, which could directly respond and adapt to changing environments to address real rather than theoretical situations. It is possible that when scaled up to architectural proportions, protocell technology could generate a sustainable, artificial reef under the foundations of Venice, by engineering a species of carbon-fixing species of light sensitive protocell. This protocell system would then be released into the canals, where it would prefer shady areas to sunlight. Protocells would be guided towards the darkened areas under the foundations of the city, which are supported by woodpiles. Here they would interact with traditional building materials and turn the foundations of Venice into stone rather than depositing their material in the lightfilled canals.14
It is envisaged that this protocell-based system would form the basis of a surface coating and repair system for existing building materials that would also be able to remove small amounts of carbon dioxide that have dissolved into the Venice lagoon from the atmosphere. With monitoring of the technology, the woodpiles would gradually become petrified; at the same time, a limestonelike reef would grow under the city, through the accretion and deposition of minerals, and would spread the point load of the buildings over a broader area, attenuating its sinking into the soft soil underneath. Simultaneously, the system could deposit solid material in any gaps forming between the buildings and their foundations. This would further stabilise the city base by extending the solid landmass around the city from the lagoon. Additionally, the reef could reduce the volume of water flowing around the city, thereby buffering it against the effects of water erosion and large movements of subterranean soil.
The issues involved with the reclamation of Venice are complex and this particular protocell-based approach addresses just one aspect of a large range of factors that threaten the continued survival of the city. The development of Living materials with programmable metabolisms suggests an entirely new approach to the production of architecture: it is possible to think about making buildings in a ‘bottom-up’ way, in a biological-like manner which is symbiotic with, and beneficial to, the environment into which these new materials are introduced.
For the successful implementation of Living materials in the built environment, appropriate procurement systems are required which, ideally, would support a broad range of synthetic biologies. Scientific research focuses exclusively on the design of the biological technology, and not at all on the supporting infrastructure and environment in which the resultant synthetic biology can exist. The built environment therefore has a critical role to play in designing meaningful applications of the spectrum of synthetic biology applications and how they are contextualised and sustained in urban contexts.15 These architectural considerations may even require a re-analysis of what constitutes a building site and how new methods of construction to support living materials are achieved.
In the near future, our buildings may facilitate our continued survival, rather than contribute to the destruction of our biosphere as they currently do. Living materials have the potential to make remedial interventions in polluted environments. They could also serve as a first line of defence against climate unpredictability. By functioning as indicators of subtle changes in our surroundings, they can act as an early warning system where subtle changes may be observed, enabling communities to take preventative action before catastrophe strikes.
Footnotes
-
‘Pakistan floods: Climate change experts say global warming could be the cause.’ Telegraph online. Accessed March 2011: http://www.telegraph.co.uk/news/worldnews/asia/pakistan/7937269/Pakistan-floodsClimate-change-experts-say-globalwarming-could-be-the-cause.html ↩
-
‘Scientists see climate change link to Australian floods.’ Accessed March 2011: http://www.dawn.com/2011/01/12/scientists-seeclimate-change-link-to-australianfloods.html ↩
-
Marilyn A. Brown, Frank Southworth and Andrea Sarzynski, ‘The geography of metropolitan carbon footprints’, Policy and Society, 27, 2009, pp. 285-304. ↩
-
United Nations, ‘Report of the World Commission on Environment and Development’. General Assembly Resolution 42/187, 11 December 1987. ↩
-
‘Sea levels rising too fast for Thames Barrier’, The Independent. Climate Change. Steve Connor, Science Editor. Saturday, 22 March 2008. Accessed February 2011: http://www.portcities.org.uk/london/server/show/ConFactFile.85/TheThames-Barrier.html ↩
-
Our Common Future, Report of the World Commission on Environment and Development: Annex, 1987. Accessed March 2011: http://www.worldinbalance.net/pdf/1987brundtland.pdf ↩
-
United Nations Environment Programme Division of Technology, Industry and Economics. Accessed March 2011: http://www.unep.or.jp/ietc/publications/freshwater/fms7/9.asp ↩
-
‘Obama’s Climate Guru, Paint your roof white’, The Independent. Accessed March 2011: http://www.independent.co.uk/environment/climate-change/obamas-climate-gurupaint-your-roof-white-1691209.html ↩
-
See: http://www.nytimes.com/2009/07/30/science/earth/30degrees.html ↩
-
N Spiller, Future City: Experiment and Utopia in Architecture: 1956-2006, Thames and Hudson, 2006. ↩
-
Mark Bedau, ‘Living Technology today and tomorrow’, Technoetic Arts, 7(2):199-206, p 199, 2009. ↩
-
R Armstrong, ‘Living Architecture’. In C Noble (Ed), Forward 110: Architecture and the Body, Spring 2010, pp 77-82, The American Institute of Architects. ↩
-
An artificial biology is a living system, or bottom-up synthetic biological system, which has been chemically self-assembled without the need for biological information molecules, such as DNA or RNA, and exhibits life-like properties such as, growth, movement or sensitivity. ↩
-
R Armstrong, ‘Living Architecture’, News in Conservation, No. 20, International Institute for Conservation, pp 4-5, 2010. ↩
-
R Armstrong and N Spiller, ‘Living Architecture’, Opinion, Nature, 467: 916–918, 2011. ↩